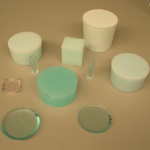
Phantoms that simulate the optical characteristics of tissues are commonly used to mimic light distributions in living tissue. Tissue phantoms are often designed and utilized for three purposes: to simulate light distributions with a geometry of physical tissue, for the calibration of optical devices, and for recording a reference measurement with an optical measurement device. For all three uses, the absorption and scattering properties of the created tissue phantom is the primary design factor. Optical tissue phantoms are necessary to calibrate steady-state optical measurements on real tissues to establish quantitative information. Optical device calibration requires using several phantoms with the optical properties that span the typical range of the tissue to be simulated. Furthermore, phantoms that are optically stable are also suitable for use as a reference measurement taken in conjunction with measurements on living tissues.
Several types of phantoms to mimic the optics of human tissue have been described in the literature including homogenized milk, non-dairy creamer, wax, and a blood and yeast suspension. Suspensions of oils/fats in an aqueous solution such as Intralipid and a water soluble dye (India ink) appear to be the most commonly used phantom materials. Difficulties arise in creating inhomogeneities using liquid phantoms such as layered structure. A desire for solid phantoms led to the development of suspensions of Intralipid and India ink in agar, agarose and polyacrylamide that could be cut and shaped to provide inhomogeneities in the phantoms. The inclusion of inhomogeneities limited the selection of dyes used to waterproof inks to prevent diffusion of dyes between regions. However, these phantoms mostly suffer from relatively short useable lifetimes which are usually limited to no more than two months.
More robust phantoms made of rubbers or plastics have been described that give long-term optical stability and greater shaping flexibility. Phantoms compositions of silicone, polyester, polyurethane, and epoxy resin have been described. There may be no great advantage of one material over another. For example, silicone more closely matches the mechanical properties of tissue and may be cast into arbitrary shapes, whereas epoxy, polyurethane, and polyesters are easier to machine after casting. Typically, the selection of a material is determined by the choice of absorbers and their stability in that medium.
The choice of scattering agents in solids is usually limited to aluminum oxide, titanium dioxide, and silicon dioxide, polyester, polystyrene, or latex microspheres. Mie theory can be used to predict the scattering properties of microspheres with knowledge of the relative refractive index, size distribution and number density. Unfortunately, microspheres tend to be much more expensive than aluminum oxide or titanium dioxide particles. One primary difference between aluminum oxide and titanium dioxide particles may be the maximum attainable value for g (the cosine for the mean angle of scattering) which describes the scattering anisotropy.
We have fabricated solid optical tissue phantoms that are castable and photostable. These optical phantoms are designed to be suitable reference standards at two distinct wavelengths and whose optical properties have been carefully measured. The final materials are verified by making several optical phantoms with differing quantities of dye and scattering particles. Phantoms made without added scatterers confirm the stability of the dyesbefore and after the curing process and over a duration of one year.
2010V. T. Keränen, A. L. Dayton, S. A. Prahl, "Polyurethane phantoms with homogeneous and nearly homogeneous optical properties," SPIE Proceedings on Design and Performance Validation of Phantoms used in Conjunction with Optical Measurement of Tissue, 7567D, 1-4 (2010).
2008A. L. Dayton, S. A. Prahl, "Turbid-polyurethane phantom for microscopy," SPIE Proceedings on Design and Performance Validation of Phantoms Used in Conjunction with Optical Measurements of Tissue, 6870, 687006-1-687006-7 (2008).
2006T. Moffitt, Y.-C. Chen, S. A. Prahl, "Preparation and characterization of polyurethane optical phantoms," Journal of Biomedical Optics, 11, 041103 (2006).
1999J. A. Viator, S. A. Prahl, "Photoacoustic Imaging of Gelatin Phantoms Using Matched Field Processing," SPIE Proceedings of Laser-Tissue Interaction X, 3601, 276-283 (1999).
J. A. Viator, S. L. Jacques, S. Prahl, "Photoacoustic Imaging of Optical Absorbers in Tissue Phantoms Using the Ray Method of Matched Field Processing," Proceedings of the Oregon Academy of Science, 35, 61 (1999 abstract only).
1997J. A. Viator, A. Shearin, S. Prahl, "Ablation Studies of Thrombus Phantoms Using a 100 μs Nd:YAG Laser at 532 nm," Proceedings of the Oregon Academy of Science, 33, 51-52 (1997 abstract only).
1996D. D. Royston, R. S. Poston, S. A. Prahl, "Optical Properties of Scattering and Absorbing Materials Used in the Development of Optical Phantoms at 1064 nm," J. Biomedical Optics, 1, 110-116 (1996).
1989C. J. M. Moes, M. J. C. van Gemert, W. M. Star, J. P. A. Marijnissen, S. A. Prahl, "Measurements and Calculations of the Energy Fluence Rate in a Scattering and Absorbing Phantom at 633 nm," Appl. Opt., 28, 2292-2296 (1989).